Lupine Publishers | Journal of Environmental & Soil Sciences
Abstract
Electricity makes our lives better, brighter, and cleaner. But before it is transmitted on high-voltage power lines and then distributed to our homes and businesses, it needs to be generated by a power plant. Electricity is vital to modern life. It powers our lights and appliances at home. It powers many industry processes. It is used to power trains and to charge electric vehicles. Globally, electricity use is rising rapidly as new major economies develop in giant countries in population. This need for electricity drives a growing demand for electricity generation, with thousands of new power plants needed across the world over the coming decades. The fundamentals of electricity generation haven’t changed much over time, but the associated technologies have radically changed over time and are constantly evolving. Politics also plays a large role and influences the types of fuels we use for electricity, and politics will continue to drive major changes in the future. Energy sources are either fossil fuels, nuclear, renewable energy. Electricity generation is a key contributor to global emissions of greenhouse gases (GHG), NOx and SO2 and their related environmental impact.
Keywords: Electricity; Electricity production; Fossil Fuel; Nuclear Power; Renewable energy
Abbreviations: AC: Alternating Current; CCGT: Combined Cycle Gas Turbines; CCS: Carbon dioxide Capture and Storage; CH4 : Methane; CO: Carbon Monoxide; CO2 : Carbon Dioxide; CSP: Concentrated Solar Power; GHG: Green House Gases; HVDC: High Voltage Direct Current; IGCC: Integrated Coal Gasification Combined Cycle; LCA: Life Cycle Assessment; LCI: Life Cycle Inventory; NH3 : Ammonia; N2 O: Nitrous Oxide; PV: Solar Photovoltaic; SO2 : Sulfur Dioxide; UF6 : Uranium Hexafluoride; UO2 :Uranium Dioxide
Introduction
Electricity plays an important role, especially in developing economies because the efficient usage of different resources depends directly on the electricity. Almost all sectors, whether it is agriculture sector, industrial sector, or household, all depend directly on electricity [1]. But Electricity generation is a key contributor to global emissions of greenhouse gases (GHG), NOx and SO2 and their related environmental impact. There are a lot of case studies including the life cycle assessment (LCA) of electricity generation depending on hard coal, lignite, natural gas, oil, nuclear, biomass, hydroelectric, solar photovoltaic (PV) and wind was studied to know the ranges of emission data for GHG, NOx and SO2 related to individual technologies [2]. With the increase in energy demand and the expected shortage of the fossil fuel with time the need for sustainable resources increases. Hence, this is initially handled by using clean fuels [3], utilization of waste heat [4-8] and adopting different configurations [9-11], where resources and environment are conserved. Between 1990 and 2008, world energy consumption increased by 40%. nowadays 68% of the energy used in the world originates from fossil fuels, with electricity generation being responsible for 40% of global CO2 emissions [12]. Between 1973 and 2007 the worldwide electricity generation increased threefold as the world became more and more dependent on electricity to meet its energy needs as shown in Figure 1.
Currently, and in the past, the supply is almost entirely based on conventional fossil fuels: they amount to worldwide over 80 percent of the generated 19,771 TWh in 2007 [13-14]. Emissions of GHG, NOx and SO2 were selected depending on their contribution to many critical LCA influence categories and on their importance in decision making and strategic planning. in all the world, the energy sector contributes 19% and 56% of overall NOx and SO2 emissions [15], respectively, however contributions to GHG emissions amount to 40%. In addition to NH3 , emissions of NOx and SO2 are largely responsible for acidification. Because NH3 is primarily emitted from animal waste in agriculture [16], NOx and SO2 emissions provide a reasonable approximation for contributions to acidification due to electricity generation. The only technology available to mitigate GHG emissions from large-scale fossil fuel usage is carbon dioxide capture and storage (CCS). CCS is a process whereby CO2 shall be captured from gases that would otherwise be emitted via smokestacks into the atmosphere, and then be injected into deep geologic formations for permanent storage [17]. However, CCS already faces many challenges that are not only related to issues such as financing demonstration projects and integration of adequate infrastructures, but also to efficiency. For example, capturing and compressing CO2 would increase the fuel needs of a coal-fired power plant by 25 to 40 percent [18-20].
Electricity is a primary energy carrier, and the emission data that are related to electricity generation are utilized extensively for accounting and reporting purposes. Datasets and emission factors for electricity generation are utilized often when performing LCA and/or GHG accounting of products. However, even though the importance of data reliability and the large number of studies that value electricity generation, significant discrepancies can be found among LCI datasets for similar electricity technologies [21-23]. Policies to decrease climate change are driving the decarburization of electricity generation worldwide and may be tackled by a combination of technologies, from renewables like hydro, wind and solar, to fossil fuels with carbon capture and storage (CCS) and nuclear power [24-26]. For example, In the UK, 90% of electricity generation generated by thermoelectric power stations, whilst electricity sector abstractions make up approximately half of all water abstractions in England and Wales [27].
For UK the electricity mix is dominated by thermoelectric generation capacity which contributes to 90% of the roughly 380 TWh generated each year. Of electricity supplied to the grid, in 2010 conventional thermal contributed 124 TWh (34%), combined cycle gas turbines (CCGT) 168 TWh (46%), nuclear 56 TWh (15%) and the remaining 17 TWh (5%) was renewables as shown in Figure 2 [28-29]. Energy has become a crucial element for sustainable development and well-being of any country in modern era. Thermoelectric generation contributes to 80% of global electricity production. Cooling of thermoelectric plants is often achieved by water abstractions from the natural environment. Globally, 80% of electricity generation generated from thermoelectric power stations such as fossil fuels and nuclear, all of it requires cooling for efficient and safe operation [30]. Most of the power in the United States produced from thermal generation-using heat to create highpressure steam and drive turbines [31]. By this coal, nuclear, and most natural gas plants produce electricity. In 2013, coal, natural gas, and nuclear power accounted for a combined 86% of total electricity generation in the U.S. (39.1% coal, 27.4% natural gas, and 19.4% nuclear) as shown in Figure 3 [32]. There are 4 main types of cooling used by the electricity sector which use different amounts of water and energy as shown in Table 1 [33]. Along many decades almost all the consumed electricity in the world has been generated from three different forms of power plant - fossil, hydro and nuclear. Renewables currently generate a relatively small share of the world’s electricity, although that share is growing fast [34].
Fossil Fuel Power Plants
Fossil fuels are an important part of global energy portfolio and play a significant role in current and future domestic energy security. A sustainable, low-carbon future will need to extract carbon dioxide from major coal and natural gas plants. However, carbon dioxide emissions from fossil fuel generation are usually very variable every day, with daily side variations between plants. We consider this change is critical to determining the appropriate carbon price as well as determining whether the CO2 power plant will capture [35-36]. Natural gas is playing an increasingly important role in the current and future world energy portfolio. For example, the ratio of the US’ electricity generated by natural gas increased from under 18% in 2002 to almost 25% in June 2012 [37], with increase of around 40% (coal use fell from 50% to 42% and non-hydro renewables rose from 2% to 5% in the same time period).More than 65% of the world’s electrical energy used today is generated by steam turbine generators burning fossil fuels as their source of energy and large scale fossil fueled plants provide most of the world’s base load generating capacity. Fossil fueled plants use either coal (60%), oil (10%) or gas (30%) in purpose designed combustion chambers to raise steam. These are all nonrenewable resources whose supply will ultimately be exhausted [38-40]. Figure 4 shows the fossil fuel powered steam turbine electricity generation.
Fossil fuel power plants burn carbon fuels such coal, oil or gas to generate steam that drives large turbines that produce electricity. These plants can generate electricity reliably over long periods of time. However, by burning carbon fuels they produce large amounts carbon dioxide, which causes climate change. They can also produce other pollutants, such as sulfurous oxides, which cause acid rain [41,42]. Fossil fuel plants require huge quantities of coal, oil or gas. These fuels may need to be transported over long distances. The price of fuels can rise sharply at times of shortage, leading to unstable generation costs [43]. In fossil-fuel power plants, water is heated by burning fossil fuels in a furnace to produce steam which drives a turbine as shown in Figure 5. The turbine is attached to an electric generator that converts mechanical energy into electricity. During this process, about 60 percent of the energy input is “lost” irreversibly [44,45]. The losses occur within:
a. The burning process (flaring losses).
b. In the conversion of liquid water to steam.
c. In heat radiation.
d. The mechanical losses in the turbine and the generator and in cooling the steam after it has been used in the turbine.
Many Other Places
The electricity generated at the power plants is alternating current (AC)at relatively low voltage, because of insolation inside the generators. The electricity is then stepped up to high voltage using transformers, before being transmitted to substations located hundreds of kilometers away [46]. Finally, the electricity enters, again via transformers, the low voltage distribution system for its use in homes, offices and factories. As an unavoidable consequence, at least another 10 percent of the electricity vanishes in transmission losses. Moreover, many of today’s electric appliances are extremely inefficient, wasting much of the power they consume as heat. Approximately 95 percent of the energy consumed by an incandescent bulb is emitted as heat, only the remaining 5 percent are given off as light [47,48]. When the entire cycle of generating, transporting and using electricity is considered, the electricity is delivered to the user of the incandescent bulb with an overall efficiency of just 2 percent.
All these losses are simply accepted because the user, be it industry or households, are paying all the losses with their utility bills [49]. If the consumers were to be shown that they are not only paying the kWh logged by their meter, but in fact the fuel which is delivered into the power plants, they might start thinking about their energy-consuming behavior [50]. Coal-fired power plants are also by far the largest source of the carbon dioxide (CO2 ) and other global-warming pollutants such as methane (CH4 ) and nitrous oxide (N2 O). The efficiency of fossil fired power plants range from 30 to 38 percent depending on the age of the plant and the technologies used [51,52]. Electricity generation from natural gas power plants changes depending on an hourly basis. Usually this is because it is easy to ramp generation up and down and send natural gas power at short notice for changing demand throughout the day unlike, say, nuclear power that tends to fulfill steady base load requirements. Figure 6 shows electricity generation (columns, primary y-axis) and capturable CO2 (solid areas, secondary y-axis) over a 24 h period for the average (median) natural gas power plant peaking at 1000 MW h [53,54].
Figure 6: Electricity generation (columns, primary y-axis) and capturable CO2 (solid areas, secondary y-axis) over a 24 h period for the average (median) natural gas power plant peaking at 1000 MW h [53].
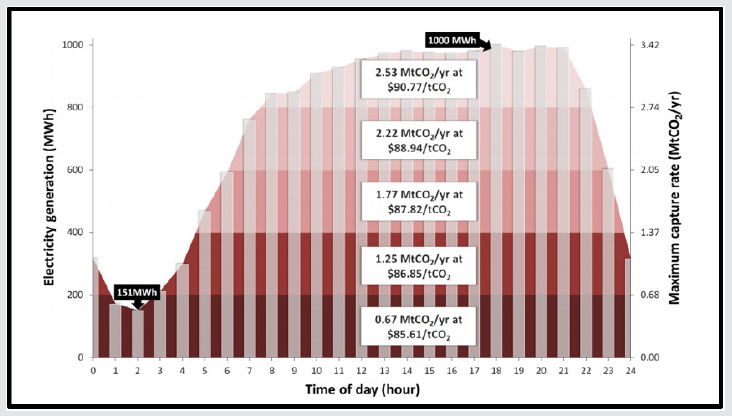
New technologies like the (ultra-) supercritical pulverized coal combustion or the process of integrated coal gasification combined cycle (IGCC) can raise the efficiency for electricity generation to 45 percent and to more than 50 percent respectively. However, the environmental damage remains the same, inducing further even unknown changes in the global climate system [55]. Fossil fuel power will continue to play an important role in local energy policy. This will be driven by relatively low-cost and abundant gas supply and demand for a clean, reliable fuel that can respond quickly to changes in the electric grid. In addition to increased use, renewable energy integration will require natural gas to generate electricity to balance fluctuating supply, increasing gas trend to be used in an uneven and peaking fashion [56].
Nuclear Power Plants
The future of nuclear power will depend on whether it can meet several objectives simultaneously economics, operating safety, proliferation safeguards and effective solutions to waste disposal. Nuclear power plants use the heat produced by nuclear fission to generate steam that drives turbines, like what happens in fossil fuel plants. However, no greenhouse gases are produced in this fission process, and only small amounts of greenhouse gases are produced across the whole fuel cycle. Nuclear power plants can run for many months without interruption, providing reliable and predictable supplies of electricity [57-60]. Some consider nuclear power plants to be a “clean” electricity source, since the plants themselves do not directly emit CO2 and other GHGs. Nevertheless, the operation of nuclear power plants results in the immense environmental impacts which are displayed in Figure 7. After a cost intensive exploration process, uranium ore is recovered from the earth’s crust under quite difficult conditions [61]. Nuclear power generation is an effective GHG mitigation option, especially by way of investments to extend the lifetime of existing plants. Whether or not building more nuclear power plants will be accepted depending on new designs becoming economically competitive, and on the industry’s ability to restore public confidence in its safe use [62].
It must be extracted from the mined ore using strong acids and bases, and then be converted into either uranium dioxide (UO2 ) for heavy water reactors or gaseous uranium hexafluoride (UF6 ) for light water reactors. Most reactors require uranium fuel to have a U-235 (an isotope of uranium) content of 3 to 5 percent. For this step, large amounts of electricity, mostly provided by fossil fuel plants, are needed to increase the actual concentration of 0.7 percent to 3 to 5 percent [63]. Afterwards, the uranium is manufactured into fuel pellets by pressing powdered UO2 or UF6 into cylindrical shapes and baking them at high temperatures, usually between 1,600 and 1,700⁰C. Finally, energy is released in a reactor by controlled nuclear fission reactions just to:
a. boil water.
b. produce steam.
c. drive a turbine that generates electricity.
This process alone has an efficiency of only 35 percent [64]. For steam production and for cooling, approximately 2.5 times more water is needed for nuclear than is required for fossil fuel plants. This is the reason why nuclear power plants are located at rivers or lakes. After the nuclear fuel is consumed in the reaction process, it is removed from the reactor and stored on site in large water-filled pools for about five years. Later, the radioactive waste is transferred to underground caverns for medium-term storage [65]. Nuclear fuel can be used in a reactor for several years. The used fuel that remains after this time must be stored and then either recycled to make new fuel or carefully disposed of. However, because the amount of fuel used to generate electricity is so much less than that used in fossil fuel plants it is much more practical to do this with used nuclear fuel than with the wastes and emissions from fossil fuels [66].
At present, there are no safe disposal facilities in operation anywhere in the world which can accept radioactive waste for permanent storage. A radioactive waste disposal facility since the seventies, its storage has recently been found to be unstable. According to World Nuclear News, roughly 126,000 barrels filled with low-level radioactive waste including contaminated clothes, paper and equipment need to be brought to the surface for alternative storage [67-69]. A challenge involves approximately Euro 3.7 billion, and a rather gracious heritage for future generation(s). Can a process like this that poses health risks exceeding that of any other process of electricity generation be called “clean”? We always need to keep in mind that already a minor failure in a nuclear power plant can create severe consequences for all forms of life on earth [70].
Renewable Energy
The negative influences of fossil fuels forced scientists to call attention to cleaner energy sources that are both friendly and renewable most suitable environment [71]. The fast depleting conventional energy sources and today’s continuously increasing energy demand in the context of environmental issues, have encouraged intensive research for new, more efficient, and green power plants with advanced technology. Since environmental protection concerns are increasing in the whole world today, both new energy and clean fuel technologies are being intensively pursued and investigated. Most of the renewable energy from wind, micro-hydro, tidal, geothermal, biomass, and solar are converted into electrical energy to be delivered either to the utility grid directly or isolated loads [72-75]. Natural energy flows vary from location to location and make the techno-economic performance of renewable energy conversion highly site specific. Intermittent sources such as wind, solar, tidal and wave energy, require backup if not grid connected, while high penetration into grids may eventually require storage and/or back-up to guarantee reliable power supply [76]. Renewable electricity has come to dominate the debate over the development of the European electricity market. Among European countries. Most wind turbines and solar panels are installed in Germany where renewable electricity has become even more important since the March 2011 decision regarding the nuclear phase-out [77]. In 2011, wind electricity accounted for 8 per cent of gross electricity production in Germany, whereas solar PV for 3 per cent. All renewable sources combined made up 20 per cent of gross electricity production in 2011 and are Germany’s second most important source of electricity generation after lignite [78]. Renewables such as wind, solar and small-scale hydro produce electricity with no greenhouse gas emissions at the point of generation and very low amounts of greenhouse gas emissions across their entire lifecycle [79]. Figure 8 shows the installed capacity and generated electricity in Germany.
Many renewables do not produce electricity predictably or consistently. Electricity generation from wind turbines varies with the wind speed. The output of solar panels is reliant on the strength of the sunshine, which depends on the time of day and the amount of cloud cover. This means that renewables have to be backed up by other forms of electricity generation, often fossil fuel generation with their resultant greenhouse gas emissions [81-83]. System and market operators in the world face two main challenges as more renewable power generation is added:
a. Electricity generated by wind turbines and photovoltaic panels is intermittent and hardly adjustable to electricity demand. Therefore, variable electricity generation is not a perfect substitute for conventional energy sources.
a. World’s renewable energy policy grants priority dispatch and fixed feed-in tariffs for renewable electricity generation. Renewable electricity can be fed into the grid whenever it is produced, regardless of energy demand, and in-feed can be switched off only if grid stability is at risk [84-85].
b. The cost of electricity generation from many renewables tends to be higher than other forms of generation. Often requiring subsidies to compete with other forms of generation, although these costs are coming down.
Wind
The global market for renewable energy sources including wind energy is growing rapidly, especially during the last two decades. The turbine has a power source fuel-free and does not cause any inexhaustible amount of pollution during the production of electricity. In addition, it can produce wind turbine energy close to load centers eliminated a transmission loss in the lines in rural and urban landscapes [86]. Wind energy is the most efficient renewable energy source for electricity generation in modern power systems. In the past decades, wind power has experienced a rapid growth worldwide. Wind power can supply up to 20% of the electricity consumption in many countries. Wind power brings many more uncertainties than conventional generation. Accurate and reliable wind power forecasting becomes extremely important to optimize the operation cost and improve the reliability of the power system with increased wind penetration [87].
While it is true that renewable energy sources are environmentally friendly, or “green”, one has also to consider their feedstock. Solar, wind, hydro and geothermal energies are “free” at first glance, although they require huge land-use investments with environmental unfriendly footprints especially biomass [88]. It is also obvious that electricity from renewable energies has considerable disadvantages in the way they are deployed today. First, and foremost, they are dependent on certain conditions (availability of wind, water and sunshine) [89]. Due to their intermitted nature, this deployment method is overstraining the grid, which is additionally rather inefficient. This fact is used by the grid operators as an argument to ask for governmental help to not only improve the grid, but to make it “smart”. At the end of 2008, the worldwide nameplate capacity of wind-powered generators added up to 121 GW, a mere of 1.5 percent of the world’s electricity usage. But the rapid growth continues, with China doubling its wind power capacity for the fifth consecutive year since 2004 [90-91].
In 2016, wind turbines in the United States were the source of nearly 6% of total U.S. utility-scale electricity generation. The amount of electricity generated from wind has grown significantly since 2000. Electricity generation from wind in the United States increased from about 6 billion (kWh) in 2000 to about 226 billion kWh in 2016. This turns out to be a big problem for the gridoperating utilities, because electricity must be used as soon as it is produced. But how easily can you forecast when and where the wind will blow? You can’t simply start a wind mill up when you need it most [92]. Thus, at least as the electricity grids are operated today, the intermittency of wind always requires backup systems with an equal amount of dispatch able generation capacity. Unfortunately, now these back-up systems are mostly conventional power plants that do not have short run-up times [93].
In addition to the unpredictability of wind, wind farms usually need high investments to be built, and are also very expensive to properly maintain. At least 20 percent of the windmills are shut off for maintenance or repairs. What is even worse, they are often taken off the grid, because their electricity is not needed at that given moment. What a system, what a waste of resources. There are no commercially viable ways to store wind energy at this time, other than pumping up water electrically in water reservoirs, but this only makes sense when wind farm and water reservoir are close to each other [94,95].
Solar
The various forms of solar energy, solar heat, solar photovoltaic, solar thermal electricity, and solar fuels offer a clean, climatefriendly, very abundant and in-exhaustive energy resource to mankind. Solar power is the conversion of sunlight into electricity, either directly using photovoltaic (PV), or indirectly using concentrated solar power (CSP) [96]. The amount of energy that comes from the sun is phenomenal: If we could somehow gather all the energy that reaches the earth on one day and store it, it would supply the energy needs of the whole world for almost 30 years [97]. Moreover, solar radiation is the sole source for fossil or renewable energy that we use today. Electricity from sunlight can be generated directly using photovoltaic, solar cells, or indirectly as with concentrating solar power. Photovoltaic systems and some other renewable energy systems are, therefore, an excellent choice in remote areas for low to medium power levels, because of easy scaling of the input power source [98,99].
Consider the interesting aspect: PV solar cells convert the sun’s radiation into DC power on which most of our appliances actually run. But this power is converted into AC power by inverters and fed into the inefficient grid, only to be inverted again to DC [100]. The efficiency of energy conversion depends mainly on the PV panels that generate power. The practical systems have low overall efficiency. This is the result of the cascaded product of several efficiencies, as the energy is converted from the sun through the PV array, the regulators, the battery, cabling and through an inverter to supply the ac load [101,102]. At this point, the most cost-effective and efficient technology for converting solar power into electricity are huge solar-thermal power plants (Figure 9). Here, sunlight is gathered by a large solar-collecting field with parabolic mirrors, so called troughs [103]. These collectors track the sun over the course of the day and concentrate the sunlight onto absorber pipes where the radiation is converted into heat. A heat transfer fluid which is circulating through the pipes is heated up to temperatures of almost 400o C [104].
The heat is used to generate steam with which electricity is then produced by conventional steam turbines. The process water is then cooled and returned to the cycle. The surplus heat could be used for heating, desalination, cooling, air conditioning, and other applications, but in most cases, it is currently rejected to the atmosphere. Solar-thermal power plants have been in commercial use for several decades since (1982). Thermal molten salt storage enables electricity production even during the night, or on cloudy days. The storage time, however, is calculated to be seven hours [105]. Water is mainly used for cooling the steam circuit, i.e. from the vaporization of water in the cooling towers. So, the plant operators not only have to capture the power of the sun, but also need immense amounts of water for cooling of the heat transfer media. As most solar power plants today is located in deserts, this physical necessity may be an obstacle to development on the long run [106].
Biomass Gasification
Gasification is a thermochemical partial oxidation process in which carbonaceous substances (biomass, coal, and plastics) are converted into gas in the presence of a gasifying agent (air, steam, oxygen, CO2 or a mixture of these). The gas generated, commonly referred to as syngas (synthesis gas), consists mainly of H2 , CO, CO2 , N2 , small particles of char (solid carbonaceous residue), ashes, tars and oils [107,108]. Gasification technology has been investigated to effectively and economically convert low value and highly distributed solid biomass to a uniform gaseous mixture mainly including hydrogen (H2 ), carbon monoxide (CO), methane (CH4 ) and carbon dioxide (CO2 ) [109]. The main target from biomass gasification process is the best possible conversion of solid biomass fuels into a high calorific product gas. Thereby, biomass reacts with air, oxygen, steam or CO2 , which provide oxygen for the process. Due to the thermal cracking and the partial oxidation a product gas is formed. The composition of the product gas depends on the biomass fuel, the reaction conditions and the fumigator and consists of different concentrations of hydrogen (H2 ), carbon monoxide (CO), steam (H2 O) and methane (CH4 ). In case of air the product gas includes nitrogen (N2 ) as well. Char coal, ash with varying carbon contents and condensable low molecular hydrocarbons are produced besides the product gas.
The char coal and the hydrocarbons (summarized as tar) are the products of an incomplete gasification [110]. Biomass is any material which once was living, and which can be utilized for energy production. The use of biomass for energy production is on rise worldwide [111]. Biomass like forest, agricultural and organic processing residues can be converted to commercial products by either biological or thermochemical processes [112,113]:
a. Biological conversion of low-value lignocellulosic biomass is facing challenges in low economy and efficiency till now [114].
b. Combustion, pyrolysis and gasification are three main thermochemical conversion methods. Biomass is traditionally burnt to supply heat and power in the process industry. The net efficiency for electricity generation from biomass combustion is usually very low, ranging from 20% to 40% [115]. Gasification provides a competitive way to convert diverse, highly distributed and low-value lignocellulosic biomass to syngas for combined heat and power generation, synthesis of liquid fuels and production of hydrogen (H2) [116-119]. But gasification is a complicated technology that’s not flexible, less competitive than others, and till now not mature so it’s exposed to certain risks plus It is not a perfect alternative for generating electricity [120].
The reasons of that:
a. It is complicated to choose the right gasifier for a given plant size, and one that is suitable for the biomass to be used.
b. There is a very wide range of designs and set-ups, many of which are still at the research stage, and biomass does not have the same steady behavior as fossil fuels.
c. Plant operation is more complex than with combustion, and it is sensitive to numerous parameters, which means that it may incur unwanted operating instabilities. Furthermore, a plant’s operating regime depends on variables that cannot always be controlled, mainly the uniformity and availability of the biomass [121-123].
Conclusion
The usage of alternating current (AC) was mainly used because AC was, at least with the technology of that time, easier to transform up to high voltage and down again. This was done in order to reduce the energy losses during transportation, especially over long distances. The latest trend is to implement high voltage direct current long distance lines (HVDC). Some of them are in daily use in India, China and Europe already, and are planned for the DESERTEC project between North Africa and Europe in 2015 or later. Daily energy losses take place in external chargers for batteries in cellular phones, laptops, cameras, navigation devices, game consoles, and electronic weather stations. In the year 2009, for example, more than 1.21 billion new mobile phones were sold worldwide and ALL of them were equipped with a new AC/DC converter to charge their batteries.
That means there are 1.21 billion of them around, only from the new cellular phones, sold in only one year. Existing AC/DC chargers are wasting our limited resources because they have to rely on an old-fashioned process of electricity production and transformation, and, in most cases also rely on an antique grid network. It seems not to be of much interest how much AC power these devices ultimate use. In fact, most of them will only deliver efficiencies in the 80 to 85 percent range. A better alternative may be to substitute DC power as a replacement for conventional AC power and thus skipping the whole power conversion process. It seems that as long as electricity remains cheap, the inefficiency of every AC/DC converter can be easily afforded. Fuel cells powered by hydrogen have the potential to do this job and thus end our reliance on global oil companies and utilities.
Acknowledgement
The author likes to acknowledge the help offered from Engineer Duaa MH Kharouf for the help during the work.
Read More About Lupine Publishers Journal of Environmental and Soil Sciences Please Click on Below Link:
https://lupine-environmental-soil-sciences.blogspot.com/