Journal of Nanomedicine| Lupine Publishers
Abstract
Keywords: Carbon Nanotubes, Photocatalytic Activity, Nanocrystals, Hybridization, Honeycomb Atomic Arrangement, Tubular Carbon Structures, Multi-Walled Carbon Nanotubes, Single-Walled Carbon Nanotubes, Atomic Vacancies, Ballistic Conduction
Introduction
CNTs have unique properties that make them potentially useful in a wide variety of applications including applications in nano-electronics, optics, and materials applications [6]. Since the beginning of the 21st century, they have been introduced in pharmacy and medicine for drug delivery system in therapeutics Because of their high surface area, excellent chemical stability, and rich electronic polyaromatic structure [7]. Also, because of unique electronic and adsorption properties of CNTs, many scientifics reported that CNTs could enhance the photocatalytic activity of semiconductors in the treatment of organic contaminants [8].
Properties of Carbon Nanotubes
Strength
CNTs have a higher tensile strength than steel. Their strength comes from the sp² bonds between the individual carbon atoms. This bond is even stronger than the sp³ bond found in diamond. Under high pressure, individual nanotubes can bond together, trading some sp² bonds for sp³ bonds. This gives the possibility of producing long nanotube wires. CNTs are not only strong, they are also elastic. You can press on the tip of a nanotube and cause it to bend without damaging to the nanotube and the nanotube will return to its original shape when the force is removed. A nanotube’s elasticity does have a limit, and under very strong forces, it is possible to permanently deform to shape of a nanotube.A nanotube’s strength can be weakened by defects in the structure of the nanotube. Defects occur from atomic vacancies or a rearrangement of the carbon bonds. Defects in the structure can cause a small segment of the nanotube to become weaker, which in turn causes the tensile strength of the entire nanotube to weaken. The tensile strength of a nanotube depends on the strength of the weakest segment in the tube similar to the way the strength of a chain depends on the weakest link in the chain [9].
Electrical Conductivity
A metallic CNT can be considered as highly conductive material. Chirality, the degree of twist of graphene sheet, determines the conductivity of CNT interconnects. Depending on the chiral indices, CNTs exhibit both metallic and semiconducting properties. The electrical conductivity of MWNTs is quite complex as their interwall interactions non-uniformly distribute the current over individual tubes. However, a uniform distribution of current is observed across different parts of metallic SWNT. Electrodes are placed to measure the conductivity and resistivity of different parts of SWNT rope. The measured resistivity of the SWNT ropes is in the order of 10−4Ωcm at 27 ͦC, indicating SWNT ropes to be the most conductive carbon fibers. It has been reported that an individual SWNT may contain defects that allows the SWNT to behave as a transistor [10].Thermal Properties
All nanotubes are expected to be very good thermal conductors along the tube, exhibiting a property known as “ballistic conduction,” but good insulators laterally to the tube axis. It is predicted that carbon nanotubes will be able to transmit up to 6000 W.m−1 .K−1 at room temperature; compare this to copper, a metal well-known for its good thermal conductivity which transmits 385 W.m−1 .K−1. The temperature stability of carbon nanotubes is estimated to be up to 2800 °C in vacuum and about 750 °C in air. Thermal expansion of CNTs will be largely isotropic, which is different than conventional graphite fibers, which are strongly anisotropic. This may be beneficial for carbon-carbon composites. It is expected that low-defect CNTs will have very low coefficients of thermal expansion [11,12].Carbon Nanotubes in Photocatalysis
Photocatalysis has been a hot topic in the degradation of organic pollutants for several decades [13]. Traditional photocatalysts include TiO2, CdS, Fe2O3, ZnO. These semiconductors suffer from some disadvantages: TiO2 has a large band gap and can only be excited by ultraviolet light, thus could not effectively harvest the spectrum of sunlight; CdS and ZnO hold the drawback of photocorrosion and cause the decrease in photoactivity and stability; in addition, all the semiconductors have the bottleneck of low quantum efficiency due to the rapid recombination of photogenerated electrons and holes, i.e., most charges quickly recombine without participating in photocatalytic reactions. Owing to their excellent mechanical, electrical and optical properties CNTs can serve as an ideal building block in hybrid catalysts and improve the performance of photocatalysts. CNT can be either semiconducting or metallic depending on their diameter and chirality. CNTs have a large electron-storage capacity and it was estimated that every 32 carbon atoms in SWCNTs can store an electron [14].When in contact with TiO2 nanoparticles, CNTs prompt electron transfer from the conducting band of TiO2 to the CNT surface due to their lower Fermi level. Thus, CNTs accept and store photogenerated electrons and inhibit the recombination of electrons and holes. Those electrons can be transferred to another electron acceptor, such as molecular oxygen, forming reactive oxygen species (O2- , H2O2 and OH) which degrade and further mineralize organic pollutants. Recent research highlighted the attraction of CNTs to TiO2 nanoparticles. CNT/TiO2 composites showed enhanced photocatalytic oxidation activity to phenol due to reduced charge recombination as evidenced by the diminished photoluminescence intensity, and SWCNT enhanced the photocatalytic activity of TiO2 better than MWCNT because there are more individual contact between the SWCNT and the TiO2 nanoparticle surface [15].
Figure 2: Proposed mechanisms of synergistic enhancement in TiO2-CNT composites: (a) CNTs inhibit charge recombination
by acting as sinks for photogenerated electrons in TiO2. (b) Photosensitizing mechanism based on electron–hole pair generation
in the CNT. Depending on the relevant positions of the bands, the electron or hole may be injected into the TiO2, generating
reactive oxygen species. (c) CNTs act as dopants through the Ti–O–C bonds.
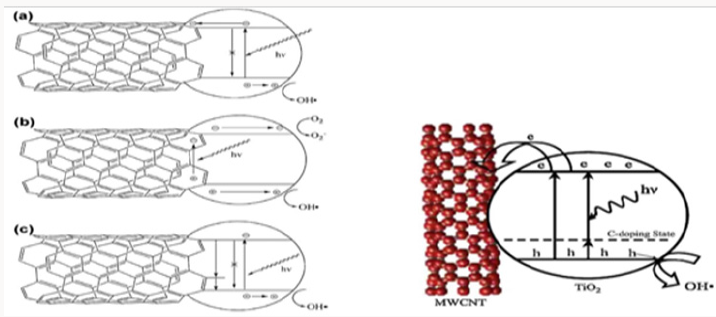
In addition to the inhabitation of charge recombination, the
introduction of CNTs increases the amount of hydroxyl groups on
the catalyst surface, which can be oxidized by h+ and generate
hydroxyl radicals [16]. Aqueous pollutants including dyes [17]
benzene derivatives [18] and carbamazepine [19] were efficiently
photodegraded by CNT-TiO2 composites. More practically, CNT/
TiO2 composite has found application in the degradation of nitro
phenols from real wastewater under sunlight and the composite
held repetitive photocatalytic activity [20]. The addition of CNT to
TiO2 may change the absorption spectrum of the catalyst. Annealing
of CNTs coated with thin and uniform TiO2 results in carbon
diffusion into oxide phase via oxygen lattice substitution. Carbon
doping produced a mid band-gap state close to the TiO2 valence
band and extended light absorption to the visible region (Figure 2)
[21]. Considering their semiconductor property, CNTs may also act
as photo-sensitizers and inject the photo-excited electrons to the
conducting band of TiO2 [22].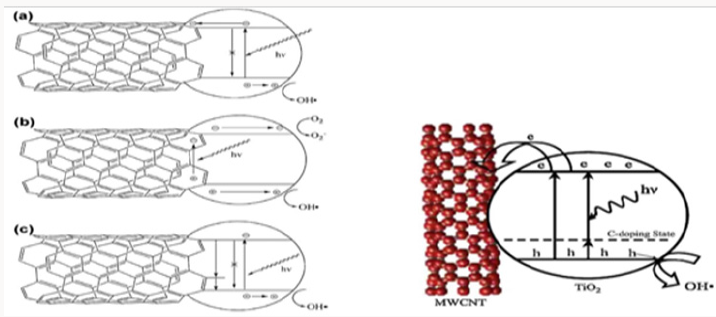
Sadeghian S [23] was synthesize titanium dioxide thin films and CNT-TiO2 nanocomposite using sol-gel method, and then characterized the samples by XRD diffraction pattern, dispersive micro-raman backscattering spectroscopy and UV-Vis absorption and transmission spectroscopies. Results show the presence of only titanium dioxide anatase phase. The preferred peak of crystal growth of TiO2 anatase is (101). X-ray diffraction pattern of CNTTiO 2 nanocomposite indicates the overlapping of (101) planes of titanium dioxide and (002) planes of carbon nanotube. By comparing the grain sizes of thin film and nanocomposite, we can see the decreasing of the particles, due to the presence of CNTs. By increasing the thickness of thin films, the grain sizes are also increased. By increasing the thickness of titanium dioxide thin films, the transmission peak shifts to the higher wavelengths. This may be due to the change in the optical energy bandgap in thicker layers. Relatively high transmission through the film indicates low roughness and high uniformity of the film’s surface.
Producing the nanocomposite, improves the crystal structure of TiO2 and we observe that the Eg peak related to anatase dioxide titanium, is sharper. By comparing UV-Vis absorption spectra of TiO2 and CNT/TiO2 nanocomposite, we can see a blue shift in absorption band of the nanocomposite. This may have occurred due to the addition of CNTs to TiO2 and decreasing in particle sizes. By addition of CNTs, we observe an increasing in absorption in visible region of light and increasing of surface charge and enhancement of photocatalytic activity of CNT/TiO2 (Figures 3-6). Appropriate synthetic approaches are needed for the improvement of the CNT/ TiO2 interface. Eder [24] used benzyl alcohol (BA) as surfactant in the preparation of CNT/TiO2 composite. The addition of BA could improve the dispersion state of TiO2 on CNTs through π-π interaction between BA and CNT and coordination between BA and titanium. Increasing the amount of BA also helped to reduce the size of the deposited TiO2 particles upon crystallization. Some studies also emphasized on the contact resistance of CNT/TiO2 in terms of electron transportation. SWCNT can enhance the photocatalytic activity of TiO2 more than MWCNT due to more individual contact, but the resistance of SWCNT is high, so there is a need to reduce the interface charge transfer resistance of SWCNT/TiO2.
CdS is a visible light-responsive photocatalyst, but it suffers from photocorrosion. Anchoring CdS onto CNTs inhibited the photocorrosion phenomenon, and the author attributed it to the enhanced adsorption capacity toward reducing agents in the solution, which can capture holes and stabilize CdS [25]. Applications of CNTs in photocatalysis also include using CNTs as pillars of reduced graphene oxide platelets for rhodamine B (RhB) degradation [26], and the preparation of Au NP@POM-CNT tricomponent hybrid photocatalyst [27]. Very interestingly, although Au nanoparticles are visible light photo-sensitizers, they don’t hold photocatalytic activity, due to the fast rate of charge recombination. The excellent electron-conducting ability of CNTs made the Au NP@POM-CNT hybrid an effective visible light photocatalyst. It is worth noting that CNTs can absorb the incident light, thus excess CNTs may have an adverse impact on the activity of the composite photocatalyst. Therefore, it is critical to control the dosage of CNTs to photocatalysts [28]. Moreover, the interfacial contact between CNTs and metallic semiconductors dictate the performance of the hybrid photocatalyst.
Figure 8: (a) Absorption spectrum of a solution of
methylene blue (MB) in the presence of pure ZnS NCs
(180mg) under exposure to UV light; (b) Absorption
spectrum of a solution of MB in the presence of ZnS/CNTs
(Zn2+-S2-, refluxing) (180mg) under exposure to UV light.
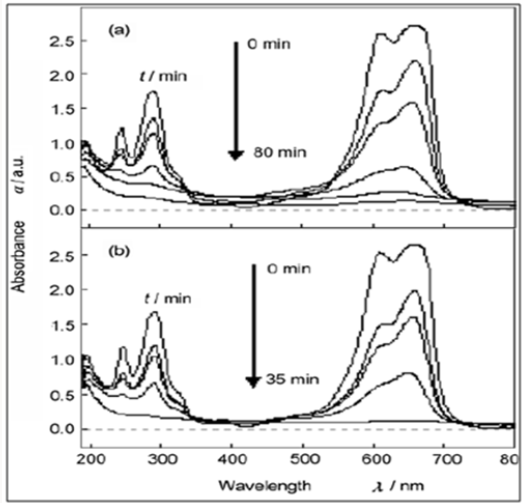
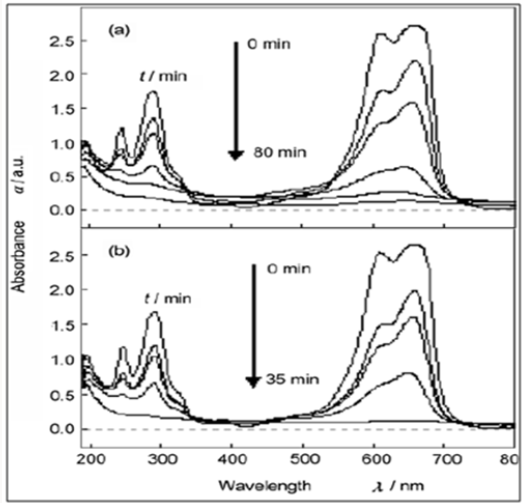
Figure 9: Conversion of MB as a function of degradation
time under exposure to UV light with different conditions:
(I) with pure ZnS NCs (180mg); (II) with ZnS/CNTs (S2-
-Zn2+) (180mg); (III) with ZnS/CNTs (S2--Zn2+, refluxing)
(180mg); (IV) with ZnS/CNTs (Zn2+-S2-, refluxing) (180mg)
and (V) with pure CNTs (180mg).

From Figure (9), the data in curves I and II, it is clear that the
photocatalytic activity of ZnS/CNTs (S2--Zn2+) is almost the same
as pure ZnS NCs. As a comparison, ZnS/CNTs (S2--Zn2+, refluxing)
shows higher photocatalytic activity, which indicates that the
interaction between CNTs and ZnS NCs is important to enhance
the photocatalytic activity of ZnS NCs. This conclusion is further
confirmed by the result that ZnS/CNTs (Zn2+-S2-, refluxing), where
the interaction between CNTs and ZnS NCs is very strong, has the
highest photocatalytic activity among these four photocatalysts.
The corresponding mechanism can be described as follows: under
UV light irradiation, the valence band electrons of ZnS are excited
and move toward its conduction bands, giving rise to the formation
of electron and hole pairs. Due to the strong interfacial connection
between ZnS NCs and CNTs, the excited e- of the conduction band
of ZnS NCs can migrate to CNTs, which are relatively good electron
acceptors [31-32], so the recombination of the e-/h+ pairs is
retarded, which results in the promotion of photocatalytic activity
in ZnS NCs. Another possible improvement of the dispersion of ZnS
NCs and thus the enhancement of the photo absorption efficiency
of ZnS NCs. This clearly suggests the relationship between the
morphology and performance of the different composite samples
used. To understand the possible contribution of MB adsorption on
CNT, associated with its tubular structure, they have performed a
blank experiment on pure CNTs. Although the employed amount
of CNTs (180mg), which is the same as that of other photocatalysts
in their experiments, is nine times that contained in the ZnS/CNTs
composite, and the reduced MB in the solution can reach only 90%
of the initial concentration, suggesting that the fast completed
elimination of ZnS/CNTs (Zn2+-S2-, refluxing) is mainly associated
with other roles rather than CNT adsorption.
Conclusion
For more Lupine
Publishers Open Access Journals Please visit our website https://lupinepublishersgroup.com/
For more Journal of Nanomedicine articles Please Click Here: https://lupinepublishers.com/nano-science-nano-technology-journal/
To Know more about Open
access journals click on Lupine
Publishers.
No comments:
Post a Comment
Note: only a member of this blog may post a comment.